Photosynthesis: A Planetary Revolution
Two and a half billion years ago, single-celled organisms called cyanobacteria harnessed sunlight to split water molecules, producing energy to power their cells and releasing oxygen into an atmosphere that had previously had none. These early environmental engineers are responsible for the life we see around us today, and much more besides.
At 8:00 p.m. on Wednesday, November 19, in Caltech's Beckman Auditorium, Professor of Geobiology Woodward "Woody" Fischer will describe how they transformed the planet. Admission is free.
Q: What do you do?
A: I'm a geobiologist of the historical variety. I'm trying to understand both how the earth works, and why it works that way. The whys are hard, because you can't redo this planetary experiment. You have to create clever ways to work backward from what you can observe to answer the question you've posed.
When you boil down the earth's history, there are maybe a half-dozen singularities—fundamental changes in how our planet and the life on it interact. Photosynthetic cyanobacteria reengineered the planet. Photosynthesis led to two more singularities—plants and animals appeared. The remaining singularities are mass extinctions as a result of something happening to the global environment, and photosynthesis likely caused one of those as well. Oxygen can be highly toxic because it's so reactive. It chews up your DNA, and it binds to the metal compounds that cells use to shuttle electrons around. Any microbes that couldn't cope with this new pollutant died off, or were forced to hide in oxygen-depleted environments.
Atmospheric oxygen resulted from a change to a microbe's metabolism that evolved once, at a specific time in the earth's history. We want to know why that happened. What were those bacteria doing beforehand? What forced them to develop this radically new way of making a living?
Bacteria don't leave fossils, per se, but they can leave behind metabolic signatures that sedimentary rocks preserve. They impact the rock's elemental composition, and they alter the ratios between heavier and lighter isotopes of certain elements as well. We can work backward from that information to deduce what the bacteria were doing on the ocean floor and in the seawater above it as those sediments were being laid down.
Q: If the earth has had breathable oxygen for billions of years, why should we care where it came from?
A: There are two really good reasons.
One has to do with meeting society's energy demands. There's a tremendous effort at Caltech and elsewhere to develop "solar fuels." Can we do better than green plants? If cyanobacteria did the best they could under tight constraints, maybe not. But if there are a variety of ways to do that chemistry, maybe we can clear the slate and do something entirely different.
The deeper reason is that atmospheric oxygen rewrote life's recipe book. Oxygen-based metabolism provides extra energy that can be invested in cellular specialization. A group of specialized cells can become a tissue, and eventually you have complex creatures with limbs. It's like agriculture—when you start growing crops, you have surplus food. Villages spring up. Craftsmen appear.
It gets to the Big Question—how rare are we? The earth is 4.5 billion years old, and the oldest evidence for life is about 3.5 billion years old. It took another billion years until photosynthesis, and two billion more for animals to develop. Is it possible to evolve advanced creatures under a different set of constraints leading to completely different metabolisms? If we're looking for life on worlds that play by different rules, will we recognize it?
Q: How did you get into this line of work?
A: As a small kid, I always loved science. That disappeared somewhere in middle school, so I went to Colorado College in Colorado Springs—a small, liberal-arts school with a really intense curriculum called the block plan. You take one class at a time for a month. You're completely immersed—lecture from nine to twelve, break for lunch, afternoon labs, evening homework. Lather, rinse, repeat. I took a geology class on a whim, because my grandfather had once taught paleontology there. The class vanished into the mountains for a month, and I was hooked.
In graduate school at Harvard, I worked with Andy Knoll, a Precambrian paleontologist who's trying to understand what the world looked like before animals. Andy's primary appointment is actually in the biology department, and I built on my sedimentary-geology background with a lot of biology classes—molecular biology, biochemistry, genomics, comparative biology, evolutionary biology. And then I came here as an Agouron Postdoctoral Scholar in Geobiology in 2007. I was fortunate that they invited me to stay.
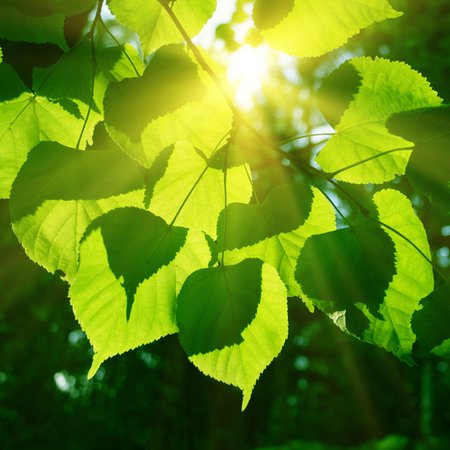
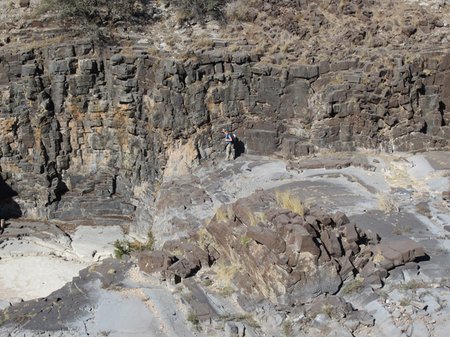
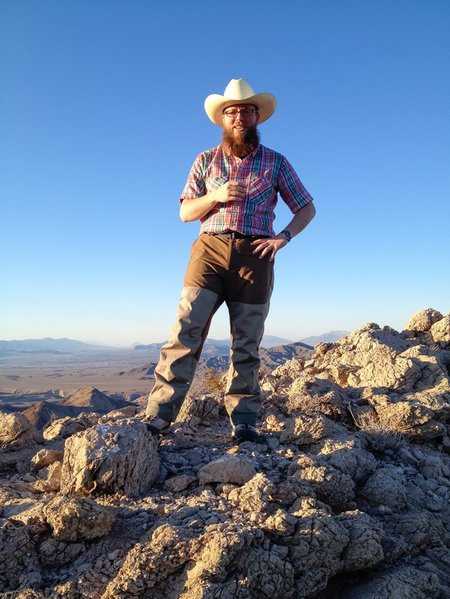
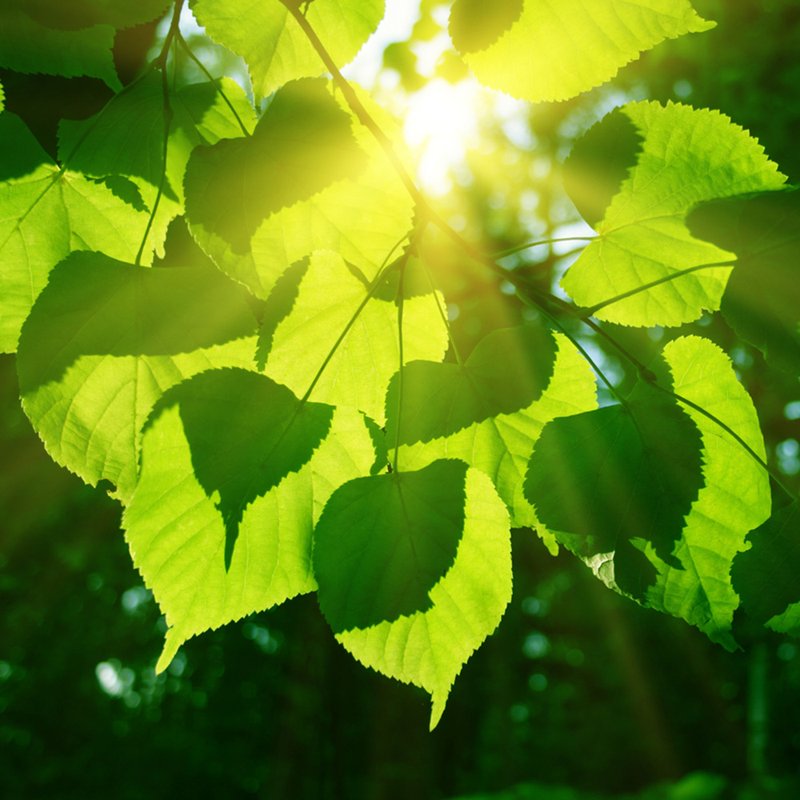
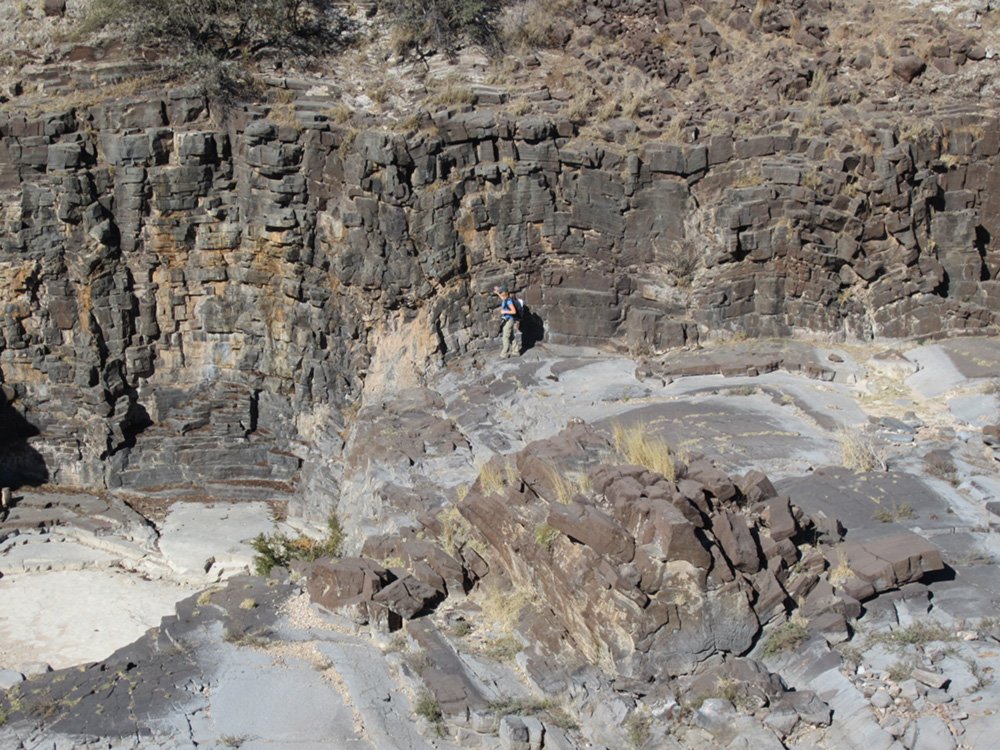
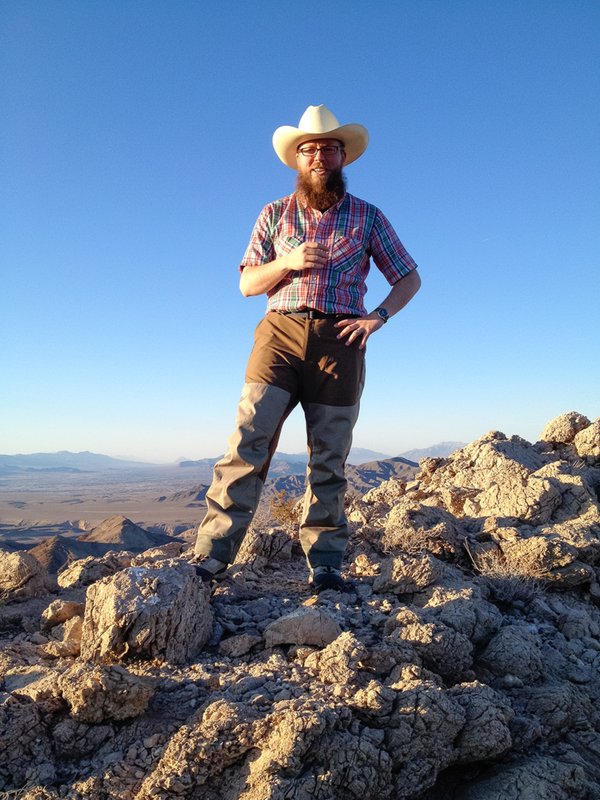